Master of Science (Biomedical Engineering) Project
I worked on designing poly (ethylene glycol)-laminin (PEG-LM) based mechanoresponsive hydrogels for drug delivery and gene therapy.
​
Project had 4 phases
1. Preliminary Report
-
Literature survey and conceptualizing previous research
-
Risk assessment and orientation
-
Experimental planning
-
Cell culture preparation
-
Day-based measurements
-
Characterization scheduling
-
-
Prepared Gantt chart for the project
2. Culture of human bone-marrow mesenchymal stem cells (hMSCs) and hydrogel preparation
-
Primary stem cells to first passage, and multiple passages based on previous viability studies
-
Hydrogel preparation through PEGylation, cross-linker and peptide chemistry, and UV photo-polymerization reaction
3. Bulk rheology and nanoindentation measurements for hMSC laden hydrogels
-
Theory and mathematics
-
Choice of parameters for characterization
-
Physical (geometry of hydrogels)
-
Instrumental (mode and technique of measurements in bulk rheology and nanoindentor)
-
Chemical (altering biodegradability through peptide (VPM)-cross-linker(4-arm acrylate PEG) ratio)
-
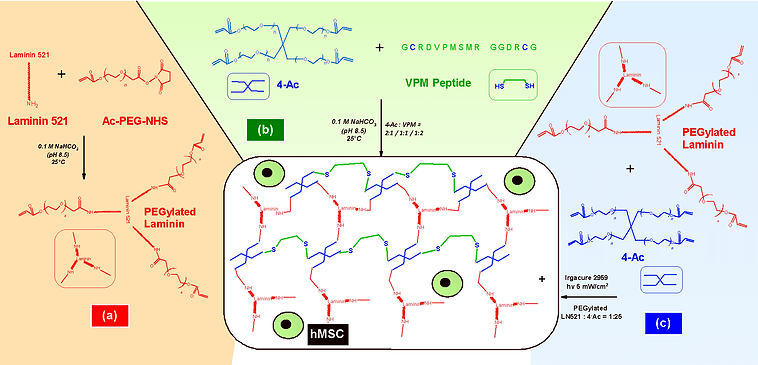
​​4. Statistical data analysis, thesis and poster submission
-
Used Prism (Graph Pad) for statistical analysis
Abstract
With advancing technology and modelling in stem cell-based tissue engineering, a strong link appears between the tissue’s mechanical properties and cellular behavior. For this, hydrogels are preferred materials for study. The focus is shifting from modelling the bio-mimetic system to the establishment of epigenetic factors that mimic embryogenesis.
​
To establish such a role, we developed three-dimensional poly(ethylene-glycol)-laminin (PEG-LM) hydrogel and incorporated it with human mesenchymal stem cells (hMSC). We varied ratios of the cross-linker (4 arm acrylate PEG or 4-Ac) to the matrix-metalloproteinase (MMP) degradable VPM peptide. This was done to tune the mechanical properties for tissue regeneration, including vascular and bone.
By using an innovative bulk rheological method and a novel nanoindentation technique, we concluded that the bulk shear elastic modulus of hydrogels is strongly dependent on the applied normal force and is independent of their chemical composition.
We also establish that
​
(1) Decreasing 4-Ac in the gel increases the rate of bio-degradation and decreases gel stiffness over time
(2) Hydrogel with a 4-Ac to VPM ratio 2 : 1 stiffens initially. This is hypothesized to be a result of the microsphere-like capability of hMSC.
(3) Presence of hMSC reduces gel stiffness regardless of the cross-linker-peptide ratio.
(4) A bi-modality in effective Young’s modulus (Eeff ) over gel area appears in nanoindentation measurements. However, more tests are required to confirm this fact.
​Poster
Thesis
References
4. Theory of elasticity / by L. D. Landau and E. M. Lifshitz. Translated from the Russian by J. B. Sykes and W. H. Reid - Details - Trove.
5. Theory of Elasticity. Theory of Elasticity (Elsevier, 1986). doi:10.1016/c2009-0-25521-8.
7. PubMed Central, Fig. 1: Matrix Biol. 2017 Jan; 57-58: 204–212. doi: 10.1016/j.matbio.2016.06.006. https://www.ncbi.nlm.nih.gov/pmc/articles/PMC5338690/figure/f0005/?report=objectonly.
8. Michael Reaction review.pdf. Wikipedia doi:nid=4169763-7.
9. Human Marrow Stromal Cells (HMSC) Culture Protocol | Sigma-Aldrich. https://www.sigmaaldrich.com/technical-documents/protocols/biology/human-marrow-stromal-cells.html.
12. Microrheology - A review - Elveflow. https://www.elveflow.com/microfluidic-tutorials/microfluidic-reviews-and-tutorials/microrheology-a-review/.
13. User Manual - Chiaro Nanoindentor. 1–148 (2012).
14. Ahearne, M. Introduction to cell-hydrogel mechanosensing. Interface Focus 4, 20130038 (2014).
15. Ahmad, N. et al. Peptide cross-linked poly (Ethylene glycol) hydrogel films as biosensor coatings for the detection of collagenase. Sensors (Switzerland) 19, 1–9 (2019).
16. Ahmed, E. M. Hydrogel: Preparation, characterization, and applications: A review. J. Adv. Res. 6, 105–121 (2015).
17. Ahn, J. Il et al. Culture of preantral follicles in poly(ethylene) glycol-based, three-dimensional hydrogel: A relationship between swelling ratio and follicular developments. J. Tissue Eng. Regen. Med. 9, 319–323 (2015).
18. Anon. MMP1 Gene - GeneCards | MMP1 Protein | MMP1 Antibody. https://www.genecards.org/cgi-bin/carddisp.pl?gene=MMP1 (2015).
19. Anton-Paar. Tips and Tricks from Joe Flow Rheometers : How and what do they measure ? Anton-Paar 1–4 (2018).
20. Baby, D. K. Rheology of hydrogels. in Rheology of Polymer Blends and Nanocomposites 193–204 (Elsevier Inc., 2020). doi:10.1016/b978-0-12-816957-5.00009-4.
21. Barros, D. et al. Engineering hydrogels with affinity-bound laminin as 3D neural stem cell culture systems. Biomater. Sci. 7, 5338–5349 (2019).
22. Bastings, M. M. C. et al. A fast pH-switchable and self-healing supramolecular hydrogel carrier for guided, local catheter injection in the infarcted myocardium. Adv. Healthc. Mater. 3, 70–78 (2014).
23. Beekmans, S. V., Emanuel, K. S., Smit, T. H. & Iannuzzi, D. Minimally Invasive Micro-Indentation: Mapping tissue mechanics at the tip of an 18G needle. Sci. Rep. 7, 1–8 (2017).
24. Butt, H. J. Controlling the flow of suspensions. Science vol. 331 (2011).
25. Caplan, A. I. & Bruder, S. P. Mesenchymal stem cells: Building blocks for molecular medicine in the 21st century. Trends Mol. Med. 7, 259–264 (2001).
26. Chan, A., Coucouvanis, E., Tousey, S., Andersen, M. & Ni, J. Improved Expansion of MSC Without Loss of Differentiation Potential. R&D systems, Inc 55413 http://www.rndsystems.com/ScientificPoster_sp_improved_expansion.aspx (2007).
27. Choi, D., Lee, W., Park, J. & Koh, W. Preparation of poly(ethylene glycol) hydrogels with different network structures for the application of enzyme immobilization. Biomed. Mater. Eng. 18, 345–356 (2008).
28. Chudoba, T. Measurement of Hardness and Young’s Modulus by Nanoindentation. in Nanostructured Coatings (eds. Cavaleiro, A. & De Hosson, J. T. M.) 216–260 (Springer New York, 2006). doi:10.1007/978-0-387-48756-4_6.
29. Ciccone, G. Multiscale Rheological Characterisation of 3-D Poly ( ethylene-glycol ) -Laminin Hydrogels For Tissue Regeneration. 74 (2019).
30. Cuy, J. L., Mann, A. B., Livi, K. J., Teaford, M. F. & Weihs, T. P. Nanoindentation mapping of the mechanical properties of human molar tooth enamel. Arch. Oral Biol. 47, 281–291 (2002).
31. Dankers, P. Y. W. et al. Development and in-vivo characterization of supramolecular hydrogels for intrarenal drug delivery. Biomaterials 33, 5144–5155 (2012).
32. Dash, R., Foston, M. & Ragauskas, A. J. Improving the mechanical and thermal properties of gelatin hydrogels cross-linked by cellulose nanowhiskers. Carbohydr. Polym. 91, 638–645 (2013).
33. de Faria, A. N., Zancanela, D. C., Ramos, A. P., Torqueti, M. R. & Ciancaglini, P. Estrogen and phenol red free medium for osteoblast culture: study of the mineralization ability. Cytotechnology 68, 1623–1632 (2016).
34. De Witte, T. M., Fratila-Apachitei, L. E., Zadpoor, A. A. & Peppas, N. A. Bone tissue engineering via growth factor delivery: From scaffolds to complex matrices. Regen. Biomater. 5, 197–211 (2018).
35. DELORY, G. E. & KING, E. J. A sodium carbonate-bicarbonate buffer for alkaline phosphatases. Biochem. J. 39, 245 (1945).
36. Elbert, D. L., Pratt, A. B., Lutolf, M. P., Halstenberg, S. & Hubbell, J. A. Protein delivery from materials formed by self-selective conjugate addition reactions. J. Control. Release 76, 11–25 (2001).
37. Eppendorf UK. Protein LoBind Tubes - Tubes, Laboratory Consumables. https://online-shop.eppendorf.co.uk/UK-en/Laboratory-Consumables-44512/Tubes-44515/Protein-LoBind-Tubes-PF-56251.html (2016).
38. Ferreira, N. N. et al. Recent advances in smart hydrogels for biomedical applications: From self-assembly to functional approaches. Eur. Polym. J. 99, 117–133 (2018).
39. Ferreira, S. A. et al. Bi-directional cell-pericellular matrix interactions direct stem cell fate. Nat. Commun. 9, 1–12 (2018).
40. Field, J. S. & Swain, M. V. Determining the mechanical properties of small volumes of material from submicrometer spherical indentations. J. Mater. Res. 10, 101–112 (1995).
41. Floren, M. et al. Human mesenchymal stem cells cultured on silk hydrogels with variable stiffness and growth factor differentiate into mature smooth muscle cell phenotype. Acta Biomater. 31, 156–166 (2016).
42. Foster, G. A. et al. Protease-degradable microgels for protein delivery for vascularization. Biomaterials 113, 170–175 (2017).
43. Foyt, D. A. et al. Hypoxia impacts human MSC response to substrate stiffness during chondrogenic differentiation. Acta Biomater. 89, 73–83 (2019).
44. Francisco, A. T. et al. Photocrosslinkable laminin-functionalized polyethylene glycol hydrogel for intervertebral disc regeneration. Acta Biomater. 10, 1102–1111 (2014).
45. Francisco, A. T. et al. Injectable laminin-functionalized hydrogel for nucleus pulposus regeneration. Biomaterials 34, 7381–7388 (2013).
46. Gäbler, S. et al. Determination of the viscoelastic properties of hydrogels based on polyethylene glycol diacrylate (PEG-DA) and human articular cartilage. Int. J. Mater. Eng. Innov. 1, 3–20 (2009).
47. Garg, K. Laminin Enriched Scaffolds for Tissue Engineering Applications. Adv. Tissue Eng. Regen. Med. Open Access 2, (2017).
48. Ge, J. et al. The Size of Mesenchymal Stem Cells is a Significant Cause of Vascular Obstructions and Stroke. Stem Cell Rev. Reports 10, 295–303 (2014).
49. Gentleman, E. et al. Comparative materials differences revealed in engineered bone as a function of cell-specific differentiation. Nat. Mater. 8, 763–770 (2009).
50. Ghica, M. V., Hîrjău, M., Lupuleasa, D. & Dinu-Pîrvu, C. E. Flow and Thixotropic Parameters for Rheological Characterization of Hydrogels. Molecules 21, (2016).
51. Gina Paroline. Basics of Applied Rheology Gina Paroline Manager of Technical and Professional Development Anton Paar USA. 21–29 www.anton-paar.com (2016).
52. Global Industry Analysts; Inc. Mesenchymal Stem Cells - Market Analysis, Trends, and Forecasts. https://www.strategyr.com/market-report-mesenchymal-stem-cells-forecasts-global-industry-analysts-inc.asp (2019).
53. Golshan, A., Curtis, J. A., Lianos, V., Rabbany, S. Y. & De Guzman, R. C. Compressive strengths of PEG gels with glycerol and bioglass particles. J. Mater. Res. 34, 1341–1352 (2019).
54. González-Cruz, R. D., Fonseca, V. C. & Darling, E. M. Cellular mechanical properties reflect the differentiation potential of adipose-derived mesenchymal stem cells. Proc. Natl. Acad. Sci. U. S. A. 109, (2012).
55. Gotro, J. Rheology of Thermosets Part 2: Rheometers. Polymer Innovation http://polymerinnovationblog.com/rheology-thermosets-part-2-rheometers/ (2014).
56. Guadix, J. A. et al. Principal criteria for evaluating the quality, safety and efficacy of hMSC-based products in clinical practice: Current approaches and challenges. Pharmaceutics 11, (2019).
57. Guvendiren, M., Lu, H. D. & Burdick, J. A. Shear-thinning hydrogels for biomedical applications. Soft Matter 8, 260–272 (2012).
58. Hamidi, M., Azadi, A. & Rafiei, P. Hydrogel nanoparticles in drug delivery. Adv. Drug Deliv. Rev. 60, 1638–1649 (2008).
59. Hellmund, K. S. & Koksch, B. Self-assembling peptides as extracellular matrix mimics to influence stem cell’s fate. Front. Chem. 7, 172 (2019).
60. Herschel, W. H. & Bulkley, R. Konsistenzmessungen von Gummi-Benzollösungen. Kolloid-Zeitschrift 39, 291–300 (1926).
61. Huebsch, N. et al. Harnessing traction-mediated manipulation of the cell/matrix interface to control stem-cell fate. Nat. Mater. 9, 518–526 (2010).
62. Ingber, D. E. Cellular mechanotransduction: Putting all the pieces together again. FASEB J. 20, 811–827 (2006).
63. Ishihara, J. et al. Laminin heparin-binding peptides bind to several growth factors and enhance diabetic wound healing. Nat. Commun. 9, 2163 (2018).
64. Jain, E., Hill, L., Canning, E., Sell, S. A. & Zustiak, S. P. Control of gelation, degradation and physical properties of polyethylene glycol hydrogels through the chemical and physical identity of the crosslinker. J. Mater. Chem. B 5, 2679–2691 (2017).
65. Jansen, L. E. et al. Zwitterionic PEG-PC Hydrogels Modulate the Foreign Body Response in a Modulus-Dependent Manner. Biomacromolecules 19, 2880–2888 (2018).
66. Joas, S., Tovar, G., Celik, O., Bonten, C. & Southan, A. Extrusion-Based 3D Printing of Poly(ethylene glycol) Diacrylate Hydrogels Containing Positively and Negatively Charged Groups. Gels 4, 69 (2018).
67. Kim, J. et al. Characterization of the crosslinking kinetics of multi-arm poly(ethylene glycol) hydrogels formed via Michael-type addition. Soft Matter 12, 2076–2085 (2016).
68. Kishida, A. & Ikada, Y. Hydrogels for Biomedical and Pharmaceutical Applications. in Polymeric Biomaterials, Revised and Expanded (ed. Dumitriu, S.) 147–160 (CRC Press, 2001). doi:10.1201/9780203904671.ch6.
69. Konieczynska, M. D. & Grinstaff, M. W. On-Demand Dissolution of Chemically Cross-Linked Hydrogels. Acc. Chem. Res. 50, 151–160 (2017).
70. Kowalski, G., Kijowska, K., Witczak, M., KuterasiÅ„ski, L. & Lukasiewicz, M. Synthesis and effect of structure on swelling properties of hydrogels based on high methylated pectin and acrylic polymers. Polymers (Basel). 11, 1–16 (2019).
71. Kumar, A., Placone, J. K. & Engler, A. J. Understanding the extracellular forces that determine cell fate and maintenance. Dev. 144, 4261–4270 (2017).
72. Langdon, S. P. Cell culture contamination: an overview. in Methods in molecular medicine vol. 88 309–317 (2004).
73. Leach, S. P. Z. and J. B. Hydrolytically degradable poly(ethylene glycol) hydrogel Biomacromolecules. Biomacromolecules 11, 1348–1357 (2011).
74. Lee, K., Silva, E. A. & Mooney, D. J. Growth factor delivery-based tissue engineering: General approaches and a review of recent developments. J. R. Soc. Interface 8, 153–170 (2011).
75. Lee, S., Tong, X. & Yang, F. Effects of the poly(ethylene glycol) hydrogel crosslinking mechanism on protein release. Biomater. Sci. 4, 405–411 (2016).
76. Li, J. & Mooney, D. J. Designing hydrogels for controlled drug delivery. Nat. Rev. Mater. 1, (2016).
77. Li, J. & Kao, W. J. Synthesis of polyethylene glycol (PEG) derivatives and PEGylated - Peptide biopolymer conjugates. Biomacromolecules 4, 1055–1067 (2003).
78. Lin, C. C. & Anseth, K. S. PEG hydrogels for the controlled release of biomolecules in regenerative medicine. Pharm. Res. 26, 631–643 (2009).
79. Lopez Hernandez, H., Grosskopf, A. K., Stapleton, L. M., Agmon, G. & Appel, E. A. Non-Newtonian Polymer–Nanoparticle Hydrogels Enhance Cell Viability during Injection. Macromol. Biosci. 19, 1–7 (2019).
80. Lumiprobe. NHS ester labeling of amino biomolecules Biomolecule. 5–6 https://www.lumiprobe.com/protocols/nhs-ester-labeling (2020).
81. Lutolf, M. P. & Hubbell, J. A. Synthesis and physicochemical characterization of end-linked poly(ethylene glycol)-co-peptide hydrogels formed by Michael-type addition. Biomacromolecules 4, 713–722 (2003).
82. Lutz, J. F. & Hoth, A. Preparation of ideal PEG analogues with a tunable thermosensitivity by controlled radical copolymerization of 2-(2-methoxyethoxy)ethyl methacrylate and oligo(ethylene glycol) methacrylate. Macromolecules 39, 893–896 (2006).
83. Lv, X., Liu, C., Shao, Z. & Sun, S. Tuning physical crosslinks in hybrid hydrogels for network structure analysis and mechanical reinforcement. Polymers (Basel). 11, (2019).
84. Maisani, M., Pezzoli, D., Chassande, O. & Mantovani, D. Cellularizing hydrogel-based scaffolds to repair bone tissue: How to create a physiologically relevant micro-environment? J. Tissue Eng. 8, 2041731417712073 (2017).
85. Mather, B. D., Viswanathan, K., Miller, K. M. & Long, T. E. Michael addition reactions in macromolecular design for emerging technologies. Prog. Polym. Sci. 31, 487–531 (2006).
86. Mattei, G. & Ahluwalia, A. A new analytical method for estimating lumped parameter constants of linear viscoelastic models from strain rate tests. Mech. Time-Dependent Mater. 23, 327–335 (2019).
87. Mattei, G., Gruca, G., Rijnveld, N. & Ahluwalia, A. The nano-epsilon dot method for strain rate viscoelastic characterisation of soft biomaterials by spherical nano-indentation. J. Mech. Behav. Biomed. Mater. 50, 150–159 (2015).
88. Mattei, G., Looze, N. & Breel, E. J. Measuring micro-mechanical properties of (bio)materials by nano-indentation. (2017).
89. Mattei, G. & Ahluwalia, A. Sample, testing and analysis variables affecting liver mechanical properties: A review. Acta Biomater. 45, 60–71 (2016).
90. McKenzie, M. et al. Hydrogel-based drug delivery systems for poorly water-soluble drugs. Molecules 20, 20397–20408 (2015).
91. Merck. Common Cell Culture Problems: Poor Cell Growth | Sigma-Aldrich. Merck KGaA, Darmstadt, Germany 1 https://www.sigmaaldrich.com/technical-documents/protocols/biology/cell-culture/poor-cell-growth.html (2019).
92. Mishra, P., Nayak, B. & Dey, R. K. PEGylation in anti-cancer therapy: An overview. Asian J. Pharm. Sci. 11, 337–348 (2016).
93. Moreau, D., Chauvet, C., Etienne, F., Rannou, F. P. & Corté, L. Hydrogel films and coatings by swelling-induced gelation. Proc. Natl. Acad. Sci. U. S. A. 113, 13295–13300 (2016).
94. Morley, L. S. D. & Filonenko-Borodich, M. Theory of Elasticity. The Mathematical Gazette vol. 51 (Butterworth-Heinemann, 1967).
95. Morpurgo, M., Veronese, F. M., Kachensky, D. & Harris, J. M. Preparation and characterization of poly(ethylene glycol) vinyl sulfone. Bioconjug. Chem. 7, 363–368 (1996).
96. Morrison, F. A. Understandig Rheology. in Understanding Rheology 137–148 (Oxford University Press, Inc., 1998).
97. Mredha, M. T. I. et al. Anisotropic tough multilayer hydrogels with programmable orientation. Mater. Horizons 6, 1504–1511 (2019).
98. Myung, D. et al. Biomimetic strain hardening in interpenetrating polymer network hydrogels. Polymer (Guildf). 48, 5376–5387 (2007).
99. Navarrete, R. O. et al. Substrate stiffness controls osteoblastic and chondrocytic differentiation of mesenchymal stem cells without exogenous stimuli. PLoS One 12, 1–18 (2017).
100. Olesiak, S. E., Sponheimer, M., Eberle, J. J., Oyen, M. L. & Ferguson, V. L. Nanomechanical properties of modern and fossil bone. Palaeogeogr. Palaeoclimatol. Palaeoecol. 289, 25–32 (2010).
101. Olszowy, P., Burns, A. & Ciborowski, P. Biomolecules. Proteomic Profiling and Analytical Chemistry (Elsevier B.V., 2013). doi:10.1016/B978-0-444-59378-8.00002-5.
102. Omidian, H. & Park, K. Hydrogels. in Fundamentals and Applications of Controlled Release Drug Delivery 75–105 (Springer US, 2012). doi:10.1007/978-1-4614-0881-9_4.
103. Optics11. Optics11 Piuma & Chiaro Nanoindenter instruments - YouTube. (Optics11 B.V. KvK/CC: 52469417 VAT: NL850459734B01 Amsterdam, NL, 2017).
104. Oyen, M. L. Nanoindentation of biological and biomimetic materials. Exp. Tech. 37, 73–87 (2013).
105. Oyen, M. L. Mechanical characterisation of hydrogel materials. Int. Mater. Rev. 59, 44–59 (2014).
106. Parhi, R. Cross-linked hydrogel for pharmaceutical applications: A review. Adv. Pharm. Bull. 7, 515–530 (2017).
107. Park, H. et al. Effect of swelling ratio of injectable hydrogel composites on chondrogenic differentiation of encapsulated rabbit marrow mesenchymal stem cells in vitro. Biomacromolecules 10, 541–546 (2009).
108. Park, Y., Lutolf, M. P., Hubbell, J. A., Hunziker, E. B. & Wong, M. Bovine Primary Chondrocyte Culture in Synthetic Matrix Metalloproteinase-Sensitive Poly(ethylene glycol)-Based Hydrogels as a Scaffold for Cartilage Repair. in Tissue Engineering vol. 10 515–522 (2004).
109. Parlato, M., Reichert, S., Barney, N. & Murphy, W. L. Poly(ethylene glycol) hydrogels with adaptable mechanical and degradation properties for use in biomedical applications. Macromol. Biosci. 14, 687–698 (2014).
110. Patterson, J. & Hubbell, J. A. Enhanced proteolytic degradation of molecularly engineered PEG hydrogels in response to MMP-1 and MMP-2. Biomaterials 31, 7836–7845 (2010).
111. Peppas, N. A., Bures, P., Leobandung, W. & Ichikawa, H. Hydrogels in pharmaceutical formulations. Eur. J. Pharm. Biopharm. 50, 27–46 (2000).
112. Phelps, E. A. et al. Maleimide cross-linked bioactive PEG hydrogel exhibits improved reaction kinetics and cross-linking for cell encapsulation and in situ delivery. Adv. Mater. 24, 64–70 (2012).
113. Pittenger, M. F. et al. Multilineage potential of adult human mesenchymal stem cells. Science (80-. ). 284, 143–147 (1999).
114. Polacheck, W. J. & Chen, C. S. Measuring cell-generated forces: A guide to the available tools. Nature Methods vol. 13 415–423 (2016).
115. Pratt, A. B., Weber, F. E., Schmoekel, H. G., Müller, R. & Hubbell, J. A. Synthetic Extracellular Matrices for in Situ Tissue Engineering. Biotechnol. Bioeng. 86, 27–36 (2004).
116. Pulido, D., Briggs, D. C., Hua, J. & Hohenester, E. Crystallographic analysis of the laminin β2 short arm reveals how the LF domain is inserted into a regular array of LE domains. Matrix Biol. 57–58, 204–212 (2017).
117. Ranganathan, N., Joseph Bensingh, R., Abdul Kader, M. & Nayak, S. K. Synthesis and Properties of Hydrogels Prepared by Various Polymerization Reaction Systems. in Cellulose-Based Superabsorbent Hydrogels (ed. Mondal, M. I. H.) 487–511 (Springer International Publishing, 2019). doi:10.1007/978-3-319-77830-3_18.
118. Rizzi, S. C. et al. Recombinant protein-co-PEG networks as cell-adhesive and proteolytically degradable hydrogel matrixes. Part II: Biofunctional characteristics. Biomacromolecules 7, 3019–3029 (2006).
119. Sarvestani, A. S., Xu, W., He, X. & Jabbari, E. Gelation and degradation characteristics of in situ photo-crosslinked poly(l-lactide-co-ethylene oxide-co-fumarate) hydrogels. Polymer (Guildf). 48, 7113–7120 (2007).
120. Spicer, C. D. Hydrogel scaffolds for tissue engineering: The importance of polymer choice. Polym. Chem. 11, 184–219 (2020).
121. Stacey, G. N. Cell culture contamination. in Methods in Molecular Biology vol. 731 79–91 (2011).
122. Storage, I. General Instructions for Culturing Rat Mesenchymal Stem Cells ( CnMSC ). Stem Cells 3–5 (2005).
123. Sun, J., Li, J., Li, C. & Yu, Y. Role of bone morphogenetic protein-2 in osteogenic differentiation of mesenchymal stem cells. Mol. Med. Rep. 12, 4230–4237 (2015).
124. Sun, M. et al. Effects of matrix stiffness on the morphology, adhesion, proliferation and osteogenic differentiation of mesenchymal stem cells. Int. J. Med. Sci. 15, 257–268 (2018).
125. Tassieri, M. Microrheology with optical tweezers: peaks & troughs. Curr. Opin. Colloid Interface Sci. 43, 39–51 (2019).
126. Tirella, A., Mattei, G. & Ahluwalia, A. Strain rate viscoelastic analysis of soft and highly hydrated biomaterials. J. Biomed. Mater. Res. - Part A 102, 3352–3360 (2014).
127. Trujillo, S. et al. Engineered full-length Fibronectin-based hydrogels sequester and present growth factors to promote regenerative responses in vitro and in vivo. bioRxiv 687244 (2019) doi:10.1101/687244.
128. Tse, J. R. & Engler, A. J. Preparation of hydrogel substrates with tunable mechanical properties. Curr. Protoc. Cell Biol. 1–16 (2010) doi:10.1002/0471143030.cb1016s47.
129. Tsou, Y. H., Khoneisser, J., Huang, P. C. & Xu, X. Hydrogel as a bioactive material to regulate stem cell fate. Bioact. Mater. 1, 39–55 (2016).
130. Van De Wetering, P., Metters, A. T., Schoenmakers, R. G. & Hubbell, J. A. Poly(ethylene glycol) hydrogels formed by conjugate addition with controllable swelling, degradation, and release of pharmaceutically active proteins. J. Control. Release 102, 619–627 (2005).
131. Wang, Z. J., Hong, W., Wu, Z. L. & Zheng, Q. Site-Specific Pre-Swelling-Directed Morphing Structures of Patterned Hydrogels. Angew. Chemie - Int. Ed. 56, 15974–15978 (2017).
132. Wanxi, Z., Tianbai, H., Jiazhen, S. & Baogong, Q. A general equation for the relationship between sol fraction and radiation dose in radiation crosslinking. Int. J. Radiat. Appl. Instrumentation. Part 33, 581–584 (1989).
133. Wiederhold, B. K., Riva, G. & Graffigna, G. White paper. Annu. Rev. CyberTherapy Telemed. 11, 7 (2013).
134. Wong, R. S. H., Ashton, M. & Dodou, K. Effect of crosslinking agent concentration on the properties of unmedicated hydrogels. Pharmaceutics 7, 305–319 (2015).
135. Yahia, Lh. History and Applications of Hydrogels. J. Biomed. Sci. 04, (2015).
136. Yang, Y. H. K., Ogando, C. R., Wang See, C., Chang, T. Y. & Barabino, G. A. Changes in phenotype and differentiation potential of human mesenchymal stem cells aging in vitro. Stem Cell Res. Ther. 9, 1–14 (2018).
137. Yao, H., Wang, J. & Mi, S. Photo processing for biomedical hydrogels design and functionality: A review. Polymers (Basel). 10, 1–27 (2017).
138. Zhou, D. et al. Biomimetic Extreme-Temperature- and Environment-Adaptable Hydrogels. ChemPhysChem 20, 2139–2154 (2019).
139. Ziemkiewicz, N. et al. Laminin-111 functionalized polyethylene glycol hydrogels support myogenic activity in vitro. Biomed. Mater. 13, (2018).
140. Zustiak, S. P. & Leach, J. B. Hydrolytically degradable poly(ethylene glycol) hydrogel scaffolds with tunable degradation and mechanical properties. Biomacromolecules 11, 1348–1357 (2010).